your current location is:Home > TechnologyHomeTechnology
The combination of cellular living robots and artificial intelligence may create real "living robots"!
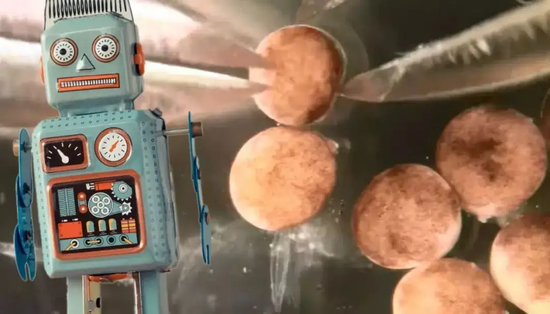
According to reports, as we learn more about how cells form and grow, the conception of the genome blue group appears to be less adequate. Scientists now point out that by combining a living robot made of cells with the exploratory capabilities of artificial intelligence, it is possible to create a purposeful "living robot"!
Where is the embryo in the human body? Embryonic morphogenesis (Morphogenesis) refers to the body formed by the embryo. The theory was once so mysterious that scholars once speculated that the body was born with tiny body structures. In the 17th century, the Dutch microscopist Nicola Ha Nicolaas Hartsoeker illustrated the scientific understanding of the "embryo preformation theory" at the time by depicting a dwarf on the top of the sperm that was stuffed into it.
Embryonic morphogenesis is expressed in the modern scientific theory that the structure of the human body is encoded in our DNA. But the more we learn about how cells generate their shape and associated morphologies, the less well-conceived the genome blueprint appears to be. Cells grow not according to genomic blueprints, if they can be thought of as programmed, not with a plan for what to make, but with a set of rules that guide what to build. One implication is that humans and other complex organisms are not the only outcome of cellular behavior, but only one of many possible outcomes.
This view of cells as contingent, constructed entities challenges our traditional theories about what the body is and what cells can form, and it also opens up some unique, even disturbing possibilities, such as: The prospect of redirecting biology into new shapes and structures means life becomes more plastic and easier to engineer and reconfigure.
An understanding of the contingency and malleability of multicellular morphology can help scientists connect the ancient evolutionary history of organisms, such as the potential benefits of being multicellular when early single-celled organisms on Earth were first discovered at the beginning of evolution. Inaki Ruiz-Trillo of the Institute of Evolutionary Biology in Barcelona, Spain said that cells may be the key to evolution, not genes or even organisms, and humans are far from reaching the tree of life. At the top, it's just that human cells have multiple functions.
Michael Levin, a biologist at Tufts University in Medford, Massachusetts, and colleagues have demonstrated that frog cells generated from normal developmental pathways can "assemble themselves" in ways that are markedly unfrog-like, the first step so far. In one of the most intriguing demonstrations so far of the cells' ability to surpass scientists' expectations, researchers isolated cells from skin cells in developing frog embryos and watched the cells move freely.
Cultivation of cells is a well-established technique in which cells are grown in a petri dish and given the nutrients they need to grow. Normally, cultured cells divide into an ever-expanding colony, but frog skin cells have other "plans." They aggregate into globular clumps of about a few thousand cells that grow on the surface of small cells called cilia. Hair-like protrusions, which are also present in the skin of normal frogs, wiggle in a coordinated and orderly fashion to propel the colony to find food, much like a rowing paddle. These clusters of cells behave themselves like tiny organisms, and may survive for a week or more, sometimes months, once food is available. The researchers, who call these cells xenobots, used skin cells from the African clawed frog (Xenopus laevis) for their experiments.
Scientists have known for more than a century that a piece of embryonic tissue destined to become skin, also known as an "animal cap", grows cilia if it is removed and nurtured. Multiple studies have shown that, given the right biochemical signals, the African clawed frog's "cap" tissue can grow into many other tissue types, such as neurons, muscles, and even beating heart tissue.
Now, Levin and colleagues point out that African clawed frog "animal cap" tissues are not just randomly formed sticky clumps of cells, they resemble autonomous organisms that, once damaged, return to their original shape, and they can also pass Pulses of calcium ions are released to send signals to each other, and while researchers aren't yet sure what those signals convey, cells move with an obvious purpose, sometimes circling each other or clumping other individual cells around them.
These cells are like LEGO versions of living robots that can be assembled in different ways!
These embryonic cells, dubbed "living robots," appear to represent a completely different developmental mechanism that frog cells can take, and that, interpreted from their usual environment, cells appear to be able to discover a new type of development, Levin said. The way of life is confusing, they are not genetically different from ordinary frog cells, so if it is not the "frog project", what role does the genome code do?
Instead, genes appear to be part of a molecular program that gives cells certain predispositions, such as sticking together in specific structures, and these cells are like Lego bricks that assemble in different ways—except for the cells themselves. In the developmental environment normal embryos experience, first they assemble to produce tadpoles and then gradually develop into frogs, but these are not the only possible solutions for cells to perform collective operations. Living robots are another form of cellular evolution, and perhaps More forms, such as certain body structures that have not yet been discovered.
Recently, Levine and colleagues discovered that living robots can exhibit a new behavior. They found that these "pseudo-organisms" can even reproduce to some extent. In experiments, they were placed in a cell culture dish and found that They move, gathering previously loose cells into piles that, in just a few days, can assemble into new living robots that move through bodily fluids, and if left to fend for themselves, they are often only able to breed a generation.
However, the researchers wondered if these mysterious cell tissues could do better, using an artificial intelligence program devised by University of Vermont researcher Josh Bonard to conduct computer simulations aimed at finding more Specializing in the structural morphology of new living robots, the results show that structures like a C-shaped half-doughnut may drive cells more efficiently than spherical living robots, forming larger spherical "progeny clusters".
The research team then manually shaped these living robots, which are about 1 millimeter in length, able to move according to computer-programmed routes, carry a certain weight, carry drugs to move inside the human body, and carry out human-impossible tasks in polluted seas. completed operation. Using tiny tools to manipulate cells into C-shaped structures and then making them work in fresh cell culture dishes, the resulting offspring will be larger than earlier living robots, and they can sustain the replication process over many more generations. The best spherical living robots can do is reproduce two generations before the offspring become too small to continue to reproduce, while C-shaped living robots can reproduce four generations, each generation is spherical, but the average size gradually decreases.
Of course, this isn't really how living organisms reproduce, living robots don't divide, so mother cells don't pass on genetic material to offspring, instead, the behavior looks more like something made of reconfigurable components Robots, which have been shown to assemble replicas of themselves when supplied with components. Living robots cannot evolve in this way, they must be endowed with components, a process that Levine and colleagues call "self-replicating kinematics" -- replicating through movement rather than biological reproduction. "Replication is for entities that can't absorb new mass themselves, they can behave, but they can't grow, and these systems can only combine matter from the external environment into copies of themselves," Bongard said.
The research shows that by combining living robots with the exploratory capabilities of artificial intelligence, it is possible to create a "living robot" with a purpose, Bongard said: "AI can be used to exaggerate a relationship with life. With the innate ability to 'program' organisms into new behaviors by rearranging biological forms rather than genes, the researchers wanted to know whether these simulated behaviors could recognize other shapes that could assemble different structures, or perhaps perform other tasks entirely. My main interest in this project is the extent to which artificial intelligence can produce living robots in ways that deviate from natural processes, and we are working on designing several new types of behaviors through artificial intelligence to incorporate into such living robots."
This view calls for a new way of thinking about cells—they are not building blocks assembled according to genetic blueprints, but entities with autonomous capabilities that can be used to make a wide variety of organisms and living structures, which one can imagine as Intelligent, reprogrammable shape-shifting robots that can move, stick together, and even send signals to each other, will be able to construct themselves into delicate "artificial objects".
This may be a better way to conceptualize how the human body is formed during embryonic development, growth is a step-by-step process, each step creates the conditions for the next, and crucially, this transition includes the state of the cell itself changes, triggered by signals from the surrounding environment, such as a layer of cells that are forced to bend as they grow in tissue that is bound to its edges by the rest of the embryo. The mechanical force at the protruding tip may be detected by sensors on the cell surface, which in turn trigger a chemical signal to the gene, after which gene activity is fired to change the properties of the cells, making them less sticky and more fluid, such as : Creates new options for the shape of body tissue. There is an "ongoing dialogue" between events taking place outside and inside the cell, as well as between the overall structural shape structure of the growing embryo and the genetic activity of internal components.
This rich and nuanced dialogue makes it difficult to predict what shape human cells will grow into. Unlike bacteria, human cells permanently alter their genetic activity as they divide and grow from general-purpose embryonic stem cells into specialized tissues. Some genes are turned off, and others are turned on, making predictions more difficult. A new discipline called "synthetic morphology" seeks to adapt, and even exploit, this complexity in order to harness the building power of cells to create entirely new, unnatural multicellular structures. If it can predict and guide outcomes, the effort will likely rely on artificial intelligence and other computing resources deployed by Levine and colleagues.
The most intriguing aspect of an organism is the overall shape structure, but this is probably the most "superficial" encoding.
Assuming that the possibility of synthetic morphologies (including the creation of entirely new organisms) is not far-fetched, living robots made of cells could be considered a biological form, despite the inconspicuousness of these tiny clumps of cells. Could frog cells, which mathematical biologists call 'attractor states' assemble computational solutions, form completely different tissues visible to the naked eye—like fish, or worms?
"We want AI to tell us more about the African clawed frog," said Bongard. "So far, two attractive features of the African clawed frog have been discovered through AI. Whether there are more secrets to the species is urgently needed. Revealed? Is there another mysterious species similar to the African clawed frog?"
This notion may go against our intuitive understanding of how life evolves, but in fact, the most striking aspect of organisms—their overall shape structure—may actually be the most “superficial” encoding, not so much the It is determined by the underlying genetic resources, rather than by the way the assembly rules happen to happen in any given situation. In a sense, the existence of tadpoles and frogs (not to mention living robots) as viable products of the African clawed frog genome supports this hypothesis - tadpoles are morphologically miniature frogs, not like babies or dwarf adults, but It is they that "run" in their own way as organisms.
Cell morphological potential reveals why humans share genetic similarities with evolutionary close relatives - with 99% genetic overlap with chimpanzees and 84% with dogs, most of human genetic material appears to be used to create and maintain human cells Build capabilities, what form did they build? From this point of view, distinguishing between human and dog bodies requires only fine-tuning of the rules governing development. Of course, these differences are critical to the evolutionary success of the ecological niche in which the organism is located, but even so, morphological It is also only insignificant in biological development research reports.
Indeed, evolutionary genetics seems to be telling us some important clues. Since the appearance of metazoans, the earliest multicellular organisms on Earth, 750 million years ago, there have not been many innovative changes in biological genes. Based on relevant data provided by the Human Genome Project, we were surprised to find that the human body encodes only as much protein as the tiny soil worm Caenorhabditis elegans, which has only 2000-3000 cells in its body. Possibly hurting human pride in a big way, maybe making people think that humans are not that special at all. This may suggest that scientists have a wrong idea of what these genetic resources are to be used for from the start—they don't provide plans, they just help organisms create conditions for selection.
After all, most of the genes and abilities needed to survive in multicellular life are even present in single-celled ancestral species, which already possessed the ability to send signals to each other to cooperate, stick together, and differentiate into different cell type. Currently, we can see this ability in the bodies of unicellular amoebae, such as the Dictyostelium discoideum mucus, which assemble into multicellular bodies when they are stressed.
Trillow and colleagues believe that the genetic resources needed for this multicellular behavior come primarily from gene regulatory mechanisms—turning them on and off—rather than from any innovation in the genes themselves. They point out that in the transition to multicellular organisms, many innovations in gene content are rooted in "tinkering" with existing gene families. The researchers came to this conclusion by studying the Capsaspora owczarzaki amoeba, one of the closest relatives in evolution to early multicellularity. It has more genes involved in regulatory functions than any other single-celled organism. Parts of the encoded proteins are called transcription factors. Trillow found that the networks of biomolecular interactions that these proteins control in Capsaspora owczarzaki are also frequently present in animals, in other words, "ready" before the rise of true multicellularity.
In a sense, tumors represent another form of our own cells.
In a sense, the human body's continued proximity to the boundary of unicellular and multicellular lifestyles may be seen as the reason why we humans (and almost all metazoans) are susceptible to cancer, in which human cells seem to have given up more The limitations required for cell life, and back to a state of excess single-cell proliferation. "Probably the most important problem in being a multicellular organism is 'tricking' some cells to make their own decisions," Trillow said. "Many animal genes that are critical to multicellular organisms are associated with cancer, and perhaps multicellular organisms are related to ancestry. The species' way of life is contradictory and requires sustained effort to maintain."
The flip side of this idea is that even single-celled organisms tend to become collective organisms, and even if cancer cells are not focused on replicating themselves, they will never ignore surrounding cells. Many cancer cells look less like undifferentiated, wildly proliferating cells and more like a disordered organ growth, and cancer cells can also differentiate and specialize, as if following some new crazy trajectory. Tumors never grow ignorant of surrounding host tissue, they bind to these tissues and even use them for their own purposes. In some ways, cancer cells represent another form of our own cells.
As multicellular life became more complex, innovative patterns of evolution in organisms continued through the application of genes rather than genes themselves, says French biologist Michel Morange: The major changes in gene regulatory networks are more the result of reorganization of gene regulatory networks than alterations in the protein links that form these networks." In 2011, developmental biologists Craig Lowe, David Hausler Haussler and their colleagues investigated what regulatory changes have been involved in the evolution of vertebrates since they first appeared 650 million years ago, comparing the genomes of various vertebrates - humans, cows, mice and two fish species (stickleback and medaka), and see which gene sequences they share, and which gene sequences their common ancestor might have had.
The researchers considered parts of the sequence that would not normally be detected in such phylogenetic comparisons -- "non-penetrant elements (CNEEs)" that lie outside the protein-coding sequence. Non-penetrant sequences are often thought of as random genomic junk that accumulates by chance, but Craig and colleagues reasoned that if some non-penetrant elements were found to be highly conserved—that is, reflected in more or less inconsistencies across species. changes, then they may play some functional role in the cell. This means that they face selection pressure that protects them, while random genomic junk degenerates rapidly and diverges sequentially across species. The researchers believe that this conserved "non-penetrant element" may be involved in regulating gene activity.
During the Cambrian explosion about 540 million years ago, a variety of animal body types appeared!
Rather than changing smoothly and incrementally, they found that animals' "non-explicit elements" appear to have undergone three distinct epochs of change since vertebrates first evolved. Until about 300 million years ago, when mammals split from birds and reptiles, changes in regulation seemed to occur primarily in parts of the genome close to transcription factors, and the key genes they control. Later, around 300-100 million years ago, these changes gradually diminished, and instead, genetic modifications were observed near genes encoded by protein molecules that act as signaling receptors on the cell surface. In other words, what is critical to these evolutionary changes is not a shift in the content of cells, but the way cells talk to each other -- the kind of dialogue that makes multicellular life possible. Finally, during the emergence of placental mammals (that is, all mammals except marsupials and monotremes such as echidnas) 100 million years ago, these regulatory changes appear to be related to mechanisms of structural modification after the protein was synthesized in its original form , especially proteins involved in transmitting signals within cells.
Evolution, then, can be thought of as, through recombination, successively discovering ways to innovate and generate new organisms, first how developmental genes are turned on and off, then how cells communicate, and finally how information is communicated within cells. In all cases, evolutionary behavior focuses on how cells interact and respond, rather than what individual cells are doing, meaning that evolution is a change in the rules of multicellular assembly. "We have about 20,000 genes that seem to be able to vary widely in shape and complexity, leading to more varied morphologies, and we think genetic complexity is governed by how many rules are encoded in the genome," Craig said. When and where genes are turned on and off, so we can explain that C. elegans, which has roughly the same number of genes as humans, remains in a low-complexity form because there are few sophisticated gene management mechanisms. "
From an evolutionary perspective, the reproductive potential of cells was evident almost as soon as multicellular construction became a lifestyle choice, and during the Cambrian explosion about 540 million years ago, a variety of bizarre Animal forms appeared, many of which were no longer seen on any living organism on Earth. To borrow Charles Darwin's "endless forms most beautiful" in On the Origin of Species, as an illustration of the potential of metazoan cell building, "endless forms most beautiful" is a description of ancestral species that could evolve into infinite forms. The possible forms refer to the many recombination schemes in the evolution of cells, which can fully express biological diversity, and at the same time, they have been strictly modified by natural selection.
Acknowledging that the human form is the accidental result of human cells being modified has raised puzzling questions, such as: Are there living human robots? If so, are they also a conceptual category of "person" despite their cellular size? Is there an organ or tissue that allows human cells to replicate, but usually has no chance of being made? Can quiescent cells in the human body "remember" earlier evolutionary body types?
"Perhaps AI algorithms can help identify some attractor elements in the ensemble of living robot types that are 'echoes' of natural selection targets," Bongard said. "At least these new structures may tell us about the environment in the distant past. And selection pressures on these organisms and how they respond evolutionarily, in a way, these attractor elements are like fossils that give us a glimpse into a part of ancient history."
At the same time, scientists are asking the question: To what extent can we reshape biological forms—including human forms? We now know that the human body has considerable "plasticity" in the sense that, for example, no genetic signal is passed on to the embryo to split into identical twins, which is just one way the rules of assembly happen to work. Even relatively modest genetic tweaks to the rules by researchers can produce markedly different body structures, such as the heritable Katagana syndrome, which causes respiratory problems in early childhood, and sometimes complete mirror-inversion of internal organs. bit. It's as if a key step in the formation of the human body's early planning goes wrong, but cells adapt as best they can, developmental problems like spina bifida, where the neural tube doesn't close to form the spinal cord, for many complex and not fully understood reasons, these Any reason can lead to the "wrong result" of the assembly rule.
Previous:How do astronauts on the International Space Station tell the difference between day and night?
Next:SpaceX wins new NASA contract: 5 new manned launch missions will be added
related articles
Article Comments (0)
- This article has not received comments yet, hurry up and grab the first frame~